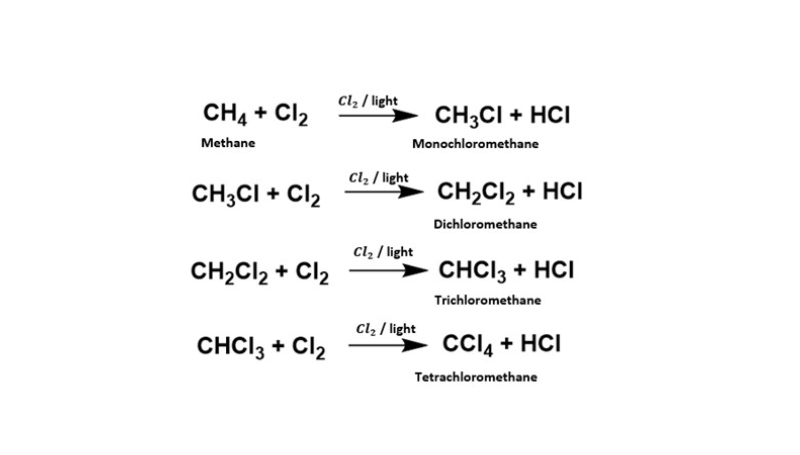
In the realm of organic chemistry, the creation of haloalkanes involves a diverse array of source materials, encompassing alkanes, alkenes, and alcohols. The predominant technique for producing these compounds involves the interaction of alcohols with hydrogen halides or phosphorus halides.
The metamorphosis of alcohols into haloalkanes unfolds through their interaction with hydrogen halides, such as hydrogen chloride (HCl), hydrogen bromide (HBr), or hydrogen iodide (HI). Termed as a nucleophilic substitution reaction, this process sees the halide ion (X-) acting as a nucleophile, directing its attack towards the carbon atom bound to the hydroxyl group (OH-). Given the hydroxyl group’s proficiency as a leaving entity, it disengages from the molecule with ease.
The reaction pace hinges on several factors, including the alcohol’s type (with primary alcohols superseding secondary ones, and tertiary alcohols lagging behind), the hydrogen halide’s type (with hydrogen iodide exhibiting greater reactivity than hydrogen bromide, which, in turn, surpasses hydrogen chloride), temperature elevation intensifying the reaction rate, and the integration of a catalyst, such as zinc chloride (ZnCl2), expediting the overall process.
Engaging with Phosphorus Halides
Another avenue for the conversion of alcohols into haloalkanes involves their reaction with phosphorus halides, such as phosphorus trichloride (PCl3) or phosphorus pentachloride (PCl5). These interactions, too, manifest as nucleophilic substitution reactions. In this scenario, the phosphorus halide adopts the role of a Lewis acid, activating the hydroxyl group and rendering it more prone to the halide ion’s assault.
Typically, the vigor of the reaction between an alcohol and a phosphorus halide eclipses that of its hydrogen halide counterpart. This heightened intensity arises from the superior reactivity inherent in phosphorus halides.
Alkenes undergo a transformation into haloalkanes through the introduction of hydrogen halides to their double bond, constituting an addition reaction. This intricate process involves the addition of a hydrogen atom from the hydrogen halide to one carbon atom of the double bond, while the halide ion adheres to the other carbon atom.
This addition process adheres to Markovnikov’s rule, dictating that the hydrogen atom affixes itself to the carbon atom of the double bond already linked to more hydrogen atoms.
The Addition of Halogens
An alternative method for converting alkenes into haloalkanes involves the addition of halogens (X2), such as chlorine (Cl2), bromine (Br2), or iodine (I2). This, too, is an addition reaction where the halogen atoms affix themselves to the two carbon atoms constituting the double bond.
In contrast to Markovnikov’s rule, this addition process is non-Markovnikov, with the halogen atoms attaching to the carbon atoms of the double bond possessing fewer hydrogen atoms.
The transition of alkanes into haloalkanes is orchestrated through free radical halogenation. This procedure involves the engagement of an alkane with a halogen gas, like chlorine (Cl2) or bromine (Br2), coupled with the presence of a free radical initiator. This initiator can take the form of a UV lamp, heat, or a chemical catalyst.
This free radical halogenation, while impactful, lacks selectivity, resulting in the formation of a haloalkane mixture, where the primary haloalkane assumes the mantle of the principal product.
Applications of Haloalkanes
Haloalkanes find diverse utility across numerous industrial and commercial domains. Prominent applications include:
- Solvents: Deployed as solvents, haloalkanes facilitate the dissolution of various substances. For instance, chlorinated solvents prove instrumental in cleansing metal surfaces.
- Refrigerants: Within refrigerators and air conditioners, haloalkanes serve as refrigerants, though the phase-out of certain variants, like chlorofluorocarbons (CFCs), ensued due to their detrimental impact on the ozone layer.
- Fire Extinguishers: Harnessing their ability to impede the combustion process, haloalkanes are integrated into fire extinguishers. Bromotrifluoromethane (CF3Br), for instance, extinguishes fires involving flammable liquids and gases.
- Pesticides: Haloalkanes contribute to pest control as pesticides, combating insects and rodents. Despite their effectiveness, some variants, such as methyl bromide (CH3Br), faced discontinuation due to environmental and health concerns.
- Pharmaceuticals: Serving as intermediates in pharmaceutical synthesis, haloalkanes play a pivotal role in crafting diverse pharmaceuticals, including corticosteroids, antibiotics, and anesthetics.